*************************************************
Intermediate mesoderm differentiates to form
kidneys, kidney ducts, and male sex ducts.
(female sex ducts develop from lateral plate mesoderm)
The most anterior intermediate mesoderm forms
the pronephros ("head kidney") (one on each side)
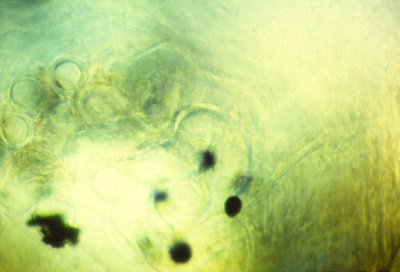
Pronephros
From each pronephros extends a pronephric duct.
The pronephric ducts connect to the cloaca, at the rear.
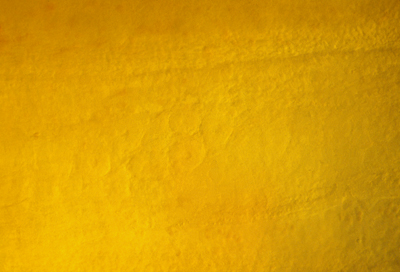
Intermediate mesoderm tubules
In amphibia all the rest of the intermediate mesoderm differentiates
into a pair (right & left) of adult kidneys, which use the pronephric duct to carry urine, and to also carry sperm in males.
But in mammals, birds and reptiles, the adult kidney develops from the most posterior parts of the intermediate mesoderm.
These adult kidneys are called the metanephros,
and each one has a special duct, called a ureter.
(the ureters form from buds off the base of the pronephric duct,
extends forward, and induces differentiation of the metanephros.
While we are embryos, we use a separate kidney, that differentiates from the middle parts of the intermediate mesoderm.
This is called the mesonephros.
Urine from it is carried to the cloaca through the pronephric duct.
Just to make things harder, the "Wolffian Duct" is a synonym for the pronephric duct, & sometimes people call it the mesonephric duct!
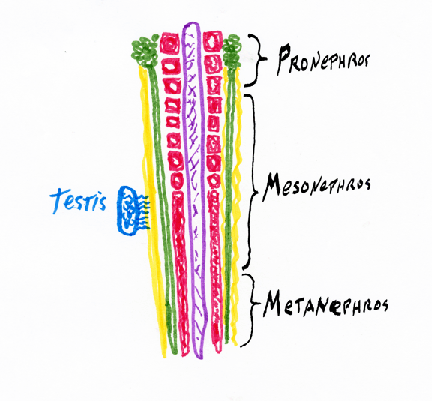
THE LECTURE ON MONDAY, FEBRUARY 20TH ENDED HERE. YOU ARE NOT RESPONSIBLE FOR ANYTHING BELOW THIS POINT ON THE SECOND HOUR EXAM
********************************************************************************
Lateral plate mesoderm splits into two layers:
(by "cavitation" + by mesenchymal cells changing into two epithelial sheets, with their apical surfaces facing each other, and fluid between them
The splanchnic layer
in contact with the endoderm below it.
The somatic layer in contact with the somatic ectoderm above it.
The space between these two layers is the coelomic cavity (=coelom),
This becomes filled with coelomic fluid, which is secreted into it by the surrounding cells.
The female sex ducts (Oviducts; Fallopian tubules, Uterus)
differentiate from outfoldings in the coelomic wall.
In Reptiles, Birds and Mammals,
the extreme right and left sides of the coelomic cavity becomes continuous with the space between the chorion and the amnion,
a fluid-filled space which is called the extraembryonic coelom.
*************************************************
The heart also develops from the extreme right and left sides of the lateral plate mesoderm;
& forms where the right & left lateral plate mesoderm come together, slightly anterior to the neck. (is its original location
In reptiles and birds, the lateral plate spreads out across the yolk, & completely surrounding the yolk, so as to form the yolk sac.
Embryos of reptiles and birds form their hearts where the right and left lateral plate mesoderm sheets come together, slightly in front of (!) where the head is forming.
Later in development, digestion of yolk causes the yolk sac to get progressively smaller, this shifts the location of the heart in a posterior direction, bringing it into the chest of the embryo.
If pieces of plastic or other physical barriers were inserted where the heart normally forms, so as to block the right and left sheets of lateral plate mesoderm from coming together !!????
What would you expect would happen?
Alternative possible experimental results!
1) No heart develops, on either side?
2) "Half-hearts" develop: incomplete structures on each side?
3) A heart develops only on the left side?
4) A heart develops only on the right side?
5) Two complete, normal hearts develop (one on each side)?
If pieces of plastic or other physical barriers were inserted where the heart normally forms, so as to block the right and left sheets of lateral plate mesoderm from coming together !!????
#5) Two complete, normal hearts develop
(one heart on each side of the barrier)
{But they are usually mirror images of each other!}
This is one of several examples in which parts of embryos can be kept apart, or mechanically split.
Another example is that if you cut a limb bud in two;
then each half can develop into a whole leg, or wing, arm, etc.
(whose muscle and bone structures are mirror images of each other)
Or (also like early embryos) you can graft two early limb buds together, and often it will develop into a single large leg, or wing, etc.
These edges of the lateral plate mesoderm can form more than two hearts, when this tissue is cut into pieces. As many as nine separate hearts were formed by one chicken embryo in some experiments done by French embryologists.
The heart has to start pumping blood very early in development,
long before the nervous system or skeletal muscles begin to function. This is because oxygen has to be carried to the tissues, and CO2 carried away. The heart beats even before the kidneys start functioning.
I want to contrast the kidney's solution to this problem of early function
in comparison with the solution in the case of the heart.
Embryos make several different pairs of kidneys, made out of different parts of the intermediate mesoderm. 3 pairs in mammals, birds & reptiles
Pronephros forming and using the pronephric duct = Wolffian Duct
Mesonephros the tubules of which connect to the Wolffian Duct
Metanephros has an entirely new and separate duct = the ureter
Your adult kidneys are your pair of metanephros kidneys
The ureter "grows" (actually its tip crawls) forward from where the Wolffian duct connects to the bladder, and because the ureter induces you most posterior intermediate mesoderm to differentiate into kidney,
a few % of people have only one kidney, from which two ureters carry urine to their bladder. If you donate a kidney, check first if you have 2
In males, the Wolffian duct becomes the sperm duct.
Mesonephric kidney tubules connect to seminiferous tubules!
The "vas deferens"
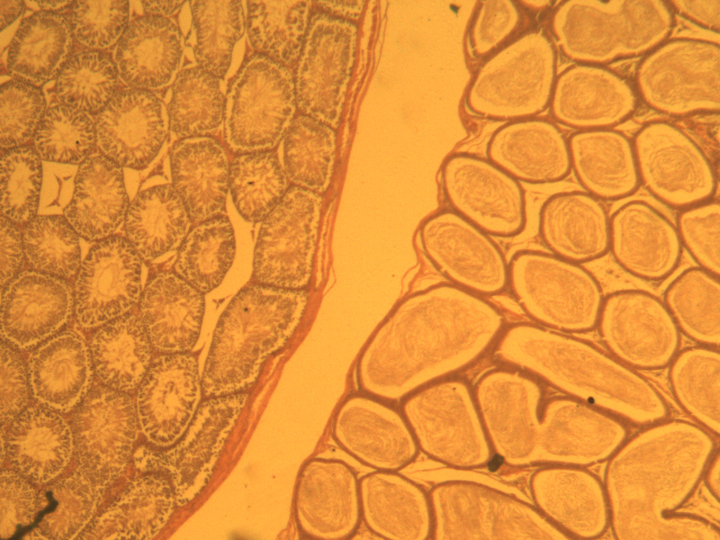
seminiferous tubules in the testis (left) and in the epididymis (right)
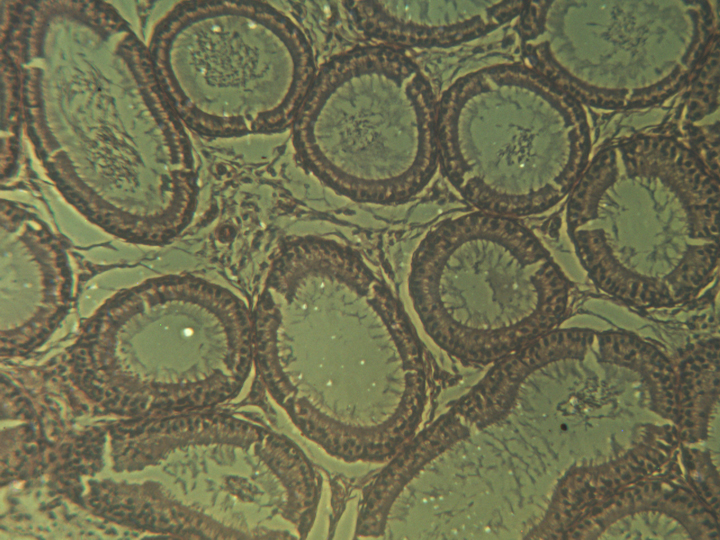
another cross-section of seminiferous tubules in the testis
In females, the Wolffian duct degenerates (apoptosis??) I don't know!
A separate "Mullerian duct" develops from lateral plate mesoderm
(in both sexes! But then the Mullerian duct degenerates in males.
The Mullerian duct becomes oviduct, fallopian tubules, & uterus.
In vertebrates, the same piece of tissue (genital ridge) differentiates into either testis or ovary.
Primordial germ cells aggregate there, guided by an unknown mechanism. These cells do NOT derive from ectoderm, mesoderm or endoderm.
nbsp;
The primordial germ cells appear brown in this photograph.
In contrast, you form one single heart (normally),
and it begins with a very simple geometric structure,
which becomes more and more complicated,
dividing into four chambers,
while beating and pumping blood the whole time!
Imagine re-building a lawn-mower motor into a 4-cycle car engine,
while the motor is running full speed the whole time!
Please notice that at the molecular level this is also astonishing,
because the shape changes are driven by special actins and myosins.
So one set of acto-myosin is driving the pulsing contraction;
and some other sets are driving the slow progressive shape changes
The heart starts as a simple peristaltic tube, with no valves and no separate chambers. It pulses from rear to front.
Later the heart then expands and gradually bends into a U shape.
Then its walls pinch inward subdividing into an atrium & ventricle.
Then a constriction pulls from front to rear,
dividing the heart into right and left atria and ventricles (four chambers)
with 4 sets of one-way valves.
This increased complexity occurs by modification and addition to the same original tissue, while the heart is beating!
"Intelligent design" might have built several different hearts,
analogous to how the kidney solves the problem of early function.
Please tell or send me your thoughts on this issue.
Before birth, blood does not need to be pumped through the lungs.
The following system for by-passing the lungs exists: until birth
There is a "trap door" opening between the right and left atria called the foramen ovale,
and also there is a short artery named the ductus arteriosus,
which connects the pulmonary artery to the aorta.
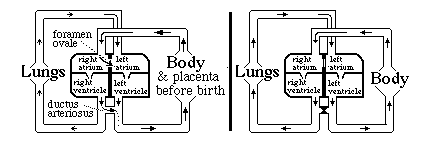
Without the ductus arteriosus, blood pumped out of the right ventricle would have no choice but to go to the lungs. Without the foramen ovale, only blood returning from the lungs would reach the left atrium.
Questions to think about and discuss in class:
a) Which direction does blood flow through the foramen ovale, prior to the moment of birth?
b) Which direction does blood flow through the ductus arteriosus before birth?
c) Why doesn't the blood flow in the other direction in the foramen ovale?
d) Why doesn't it flow in the other direction in the ductus arteriosus?
When the lungs expand and fill with air, that reduces the mechanical resistance to blood flowing through the capillaries:
How should that change the relative pressures in left versus right atria?
e) What about the relative amounts of pressure in the aorta versus the pulmonary artery, before and after the lungs expand at birth.
f) The thickness and strength of the cardiac muscle walls of the right and left ventricles are the same before birth! But after birth, the muscles of the left wall gradually become about four-times thicker and stronger on the left side than the right side? What does that tell you?
Normally (in mammals) the tissues fuse together in both the ductus arteriosus and the foramen ovale, closing both permanently.
In as many as 25% of people, some small hole remains between the atria. (without any symptoms)
Many kinds of serious birth defects in human hearts result from improper formation of the interventricular septum (septal defects).
Sometimes the ventricles remain connected (not separated)
Sometimes the aorta or the pulmonary artery are connected to the WRONG ventricle!
Such "blue babies" can develop up to the time of birth, more or less "normally", but then have severe problems
Surgery can fix most of these problems; but it is one of the most difficult areas of surgery, I have been told.
Circulatory System
The special kind of epithelial cells that line all blood vessels are called endothelial cells.
The narrowest blood vessels are called capillaries, and their walls are made of endothelial cells only (one cell layer thick; and with few or no smooth muscle cells of fibroblasts to provide mechanical reinforcement or control constriction.
Arteries are made of layers of smooth muscle cells (and some fibroblasts) and type I collagen fibers wrapped around their capillary cells.
Veins have fibroblasts and collagen wrapping the endothelial tube that lines where the blood cells are.
The first beginnings of the vertebrate circulatory system are a series of unconnected endothelial sacks, called blood islands, with red blood cells and white blood cells differentiating inside; most of these are in the yolk sac. Their endothelial cells crawl and connect to each other to form hollow tubes: the first capillaries. Only much later does bone marrow become the place where blood formation occurs; early embryos don't have bones; and when the skeleton does form it is nearly all made out of cartilage, which is solid, without a marrow, and isn't even penetrated by blood vessels (i.e. NOT vascularized) which is what you call it when these endothelial cells penetrate a tissue and form a system of capillaries, and later arteries & veins. Early embryos do not have any bone marrow yet, because nearly all their "bones" are still made of cartilage, and are solid inside. The yolk sac is one of several different organs that are used as locations for the hemopoietic stem cells which form red blood cells (and also white blood cells). These stem cells move to the liver, and later to the bone marrow.
Embryonic and fetal hemoglobins are different from (and coded for by different genes than) the hemoglobin we have in the red blood cells we make after birth. Fetal hemoglobin binds oxygen slightly more strongly than adult hemoglobin, which increases the amount of oxygen that is transferred across the placenta from the blood of a pregnant woman to the blood of the fetus developing inside her.
The genes for embryonic and fetal hemoglobin proteins are directly adjacent to the genes for adult hemoglobins, with the early blood cells activating one gene, and then later blood cells activating the gene next to that one. The mechanism isn't known, nor is it known whether there is any similarity to the mechanisms that cause adjacent Hox genes to be transcribed in adjacent tissues.
A few people continue to make fetal hemoglobin all their life, instead of switching to the adult hemoglobin genes, and the symptoms of this are almost unnoticeable! A new treatment for victims of sickle-cell anemia (and other genetic defects of adult hemoglobins), works by simulating hemopoietic stem cells back to transcribing the genes for fetal hemoglobin.
The geometry of the early vertebrate circulatory system is organized much like that of our distant fish ancestors, with blood being pumped front-ward out of the heart and around the sides of the neck in a series of 6 paired arteries like those used to provide blood to the 6 pairs of gills in a fish. Even birds and mammals form these aortic arches, even though there are no actual gills; they are in the places where gills would be. In mammals, the fourth left aortic arch becomes the aorta.
The vertebrate circulatory system begins as a series of unconnected endothelial sacks, called blood islands.
Most of these are in the yolk sac, in birds and mammals.
Maybe that's why mammal embryos continue to have yolk sacs?
Red blood cells and white blood cells differentiate inside;
Endothelial cells crawl and connect other to form hollow tubes: the first capillaries. Only much later does bone marrow become the place where blood formation occurs
Early embryos don't have bones; and when the skeleton does form it is nearly all made out of cartilage, which is solid, without a marrow, and isn't penetrated by blood vessels ("vascularized")
The yolk sac is one of several different organs that are used as locations for the hemopoietic stem cells which form red blood cells (and also white blood cells). These stem cells move to the liver, and later to the bone marrow.
|